For centuries, the intriguing question of our universe's origin was the domain of philosophers, theologians, and poets. However, during the 20th century, advancements in physics and astronomy transformed this query into a subject of empirical science.
After much deliberation and evidence gathering, the fusion of cosmic expansion, the early abundance of light elements, the universe's large-scale structure, and the cosmic microwave background collectively pointed towards the Big Bang as the fiery, dense, and expanding genesis of our contemporary universe. It wasn't until the mid-1960s that the cosmic microwave background was detected, surprisingly, it could have been discerned on an ordinary television set.
To fully grasp this phenomenon, we must comprehend the nature of the cosmic microwave background. A close look at our universe today reveals that it's teeming with galaxies - approximately 2 trillion observable ones based on current estimations. The neighboring galaxies resemble ours, housing stars similar to those in our own galaxy.
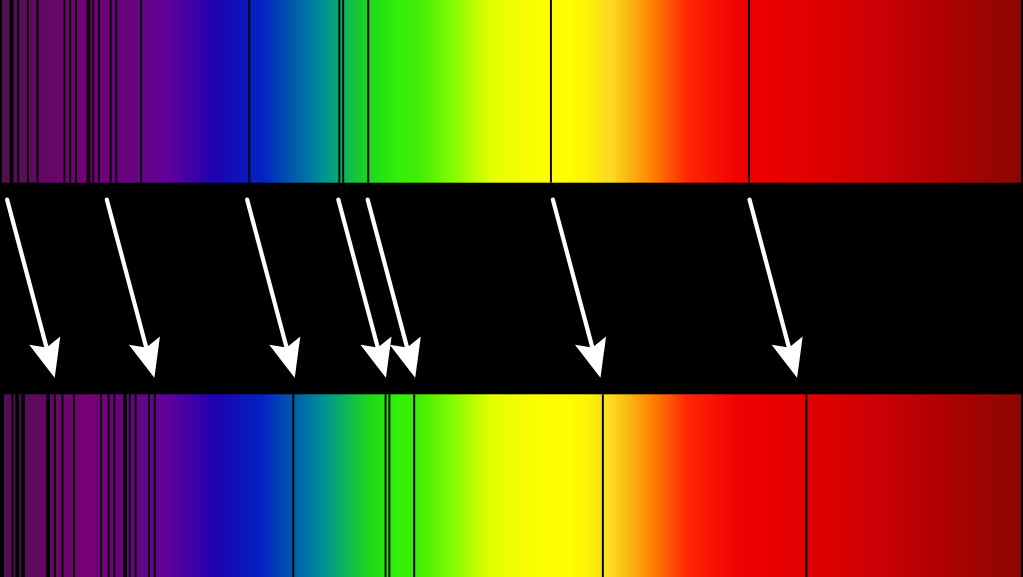
Every element in the Universe has its own unique set of atomic transitions that are allowed, corresponding to a particular set of spectral lines. We can observe these lines in galaxies other than our own, but although the pattern is the same, the lines we observe are systematically shifted relative to the lines we create with atoms on Earth.(Credit: Georg Wiora (Dr. Schorsch)/Wikimedia Commons)
Although there were many possible explanations for this observation, different ideas would give rise to different specific observable signatures. The light could be scattering off of intervening matter, which would redden it but also blur it, yet distant galaxies appear just as sharp as nearby ones. The light could be shifted because these galaxies were speeding away from a giant explosion, but if so, they would be sparser the farther away we get, yet the density of the Universe remains constant. Or the fabric of space itself could be expanding, where the more distant galaxies simply have the light shift by greater amounts as it travels throughout an expanding Universe.
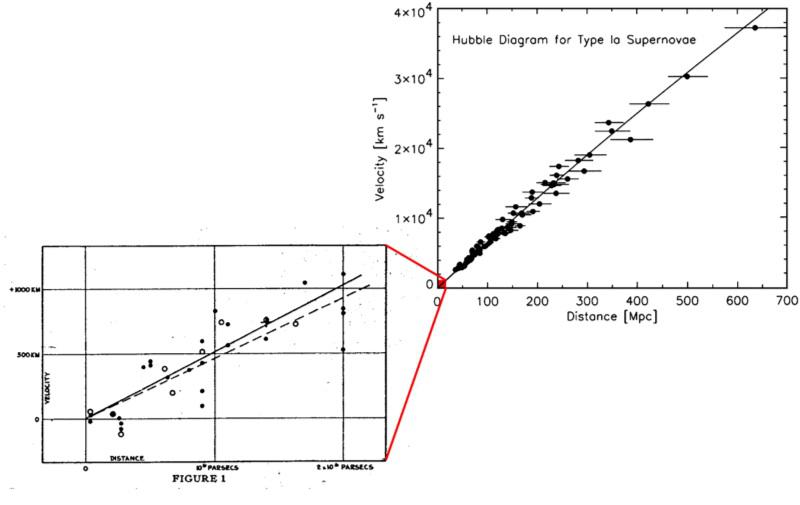
The original 1929 observations of the Hubble expansion of the Universe, followed by subsequently more detailed, but also uncertain, observations. Hubble’s graph clearly shows the redshift-distance relation with superior data to his predecessors and competitors; the modern equivalents go much farther. Note that peculiar velocities always remain present, even at large distances, but that the general trend relating distance to redshift is the dominant effect.(Credit: Edwin Hubble (L), Robert Kirshner (R))
This last point turned out to be in spectacular agreement with our observations, and helped us understand that it was the fabric of space itself that was expanding as time progresses. The reason the light is redder the farther away we look is because of the fact that the Universe has expanded over time, and the light within that Universe gets its wavelength stretched by the expansion. The longer the light has been traveling, the greater the redshift due to the expansion.
As we move forward in time, emitted light gets shifted to larger wavelengths, which have lower temperatures and smaller energies. But that means if we view the Universe in the opposite fashion — by imagining it as it was farther back in time — we’d see light that had smaller wavelengths, with higher temperatures and greater energies. The farther back you extrapolate, the hotter and more energetic this radiation should get.
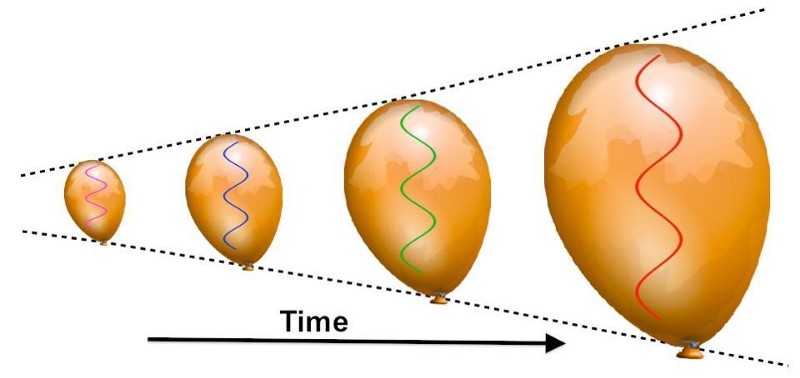
As the fabric of the Universe expands, the wavelengths of any radiation present will get stretched as well. This applies just as well to gravitational waves as it does to electromagnetic waves; any form of radiation has its wavelength stretched (and loses energy) as the Universe expands. As we go farther back in time, radiation should appear with shorter wavelengths, greater energies, and higher temperatures.(Credit: E. Siegel/Beyond the Galaxy)
Although it was a breathtaking theoretical leap, scientists (beginning with George Gamow in the 1940s) began extrapolating this property back farther and farther, until a critical threshold of a few thousand Kelvin was reached. At that point, the reasoning went, the radiation present would be energetic enough that some of the individual photons could ionize neutral hydrogen atoms: the building block of stars and the primary contents of our Universe.
When you transitioned from a Universe that was above that temperature threshold to one that was below it, the Universe would go from a state that was filled with ionized nuclei and electrons to one that was filled with neutral atoms. When matter is ionized, it scatters off of radiation; when matter is neutral, radiation passes right through those atoms. That transition marks a critical time in our Universe’s past, if this framework is correct.
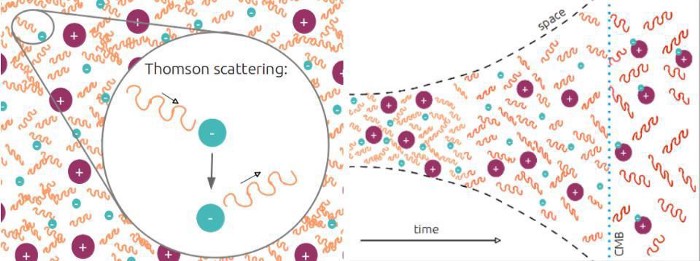
In the hot, early Universe, prior to the formation of neutral atoms, photons scatter off of electrons (and to a lesser extent, protons) at a very high rate, transferring momentum when they do. After neutral atoms form, owing to the Universe cooling to below a certain, critical threshold, the photons simply travel in a straight line, affected only in wavelength by the expansion of space.(Credit: Amanda Yoho for Starts With A Bang)
The spectacular realization of this scenario is that it means that today, that radiation would have cooled from a few thousand Kelvin to just a few degrees above absolute zero, as the Universe must have expanded by anywhere from a factor of hundreds to a few thousand since that epoch. It should remain even today as a background coming to us from all directions in space. It should have a specific set of spectral properties: a blackbody distribution. And it should be detectable somewhere in the range of microwave to radio frequencies.
Remember that light, as we know it, is much more than just the visible portion our eyes are sensitive to. Light comes in a variety of wavelengths, frequencies, and energies, and that an expanding Universe doesn’t destroy light, it simply moves it to longer wavelengths. What was ultraviolet, visible and infrared light billions of years ago becomes microwave and radio light as the fabric of space stretches.

The size, wavelength and temperature/energy scales that correspond to various parts of the electromagnetic spectrum. You have to go to higher energies, and shorter wavelengths, to probe the smallest scales. Ultraviolet light is sufficient to ionize atoms, but as the Universe expands, light gets systematically shifted to lower temperatures and longer wavelengths.(Credits: NASA and Inductiveload/Wikimedia Commons)
It wasn’t until the 1960s that a team of scientists sought to actually detect and measure the properties of this theoretical radiation. Over at Princeton, Bob Dicke, Jim Peebles (who won 2019’s Nobel Prize), David Wilkinson and Peter Roll planned to build and fly a radiometer capable of searching for this radiation, with the intent of confirming or refuting this hitherto untested prediction of the Big Bang.
But they never got the chance. 30 miles away, two scientists were making use of a new piece of equipment — a giant, ultra-sensitive, horn-shaped radio antenna — and were failing to calibrate it over and over. While signals emerged from the Sun and the galactic plane, there was an omnidirectional noise they simply couldn’t get rid of. It was cold (~3 K), it was everywhere, and it wasn’t a calibration error. After communication with the Princeton team, they realized what it was: it was the leftover glow from the Big Bang.
Subsequently, scientists went on to measure the entirety of the radiation associated with this cosmic microwave background signal and determined that it did, indeed, match the predictions of the Big Bang. In particular, it did follow a blackbody distribution, it was peaked at 2.725 K, it extended into both the microwave and radio portions of the spectrum, and it is perfectly even throughout the Universe to better than 99.99% precision.
If we take a modern view of things, we know now that the cosmic microwave background radiation — the radiation that confirmed the Big Bang and caused us to reject all the alternatives — could have been detected in any of a whole slew of wavelength bands, if only the signals had been collected and analyzed with a view toward identifying it.
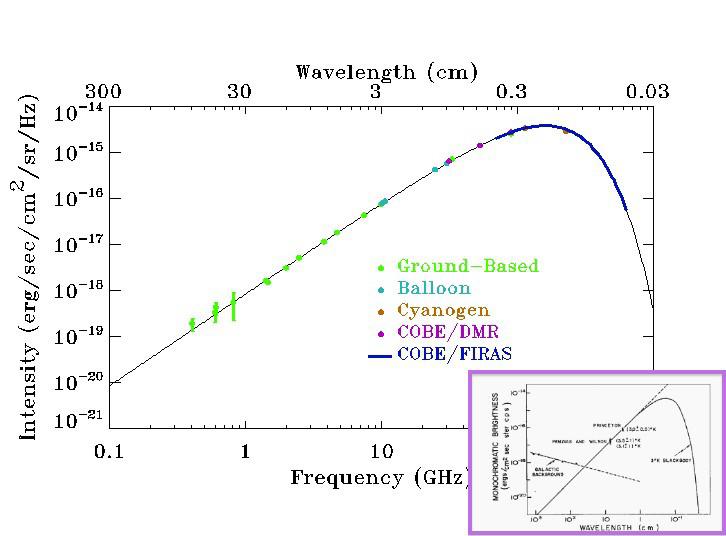
The unique prediction of the Big Bang model is that there would be a leftover glow of radiation permeating the entire Universe in all directions. The radiation would be just a few degrees above absolute zero, would be the same magnitude everywhere, and would obey a perfect blackbody spectrum. These predictions were borne out spectacularly well, eliminating alternatives like the Steady State theory from viability.(Credit: NASA/GSFC/COBE team (main); Princeton group, 1966 (inset))
Remarkably, a simple but ubiquitous device began showing up in households all over the world, particularly in the United States and Great Britain, in the years immediately following World War II: the television set.
The way a television works is relatively simple. A powerful electromagnetic wave is transmitted by a tower, where it can be received by a properly sized antenna oriented in the correct direction. That wave has additional signals superimposed atop it, corresponding to audio and visual information that had been encoded. By receiving that information and translating it into the proper format (speakers for producing sound and cathode rays for producing light), we were able to receive and enjoy broadcast programming right in the comfort of our own homes for the first time. Different channels broadcasted at different wavelengths, giving viewers multiple options simply by turning a dial.
Unless, that is, you turned the dial to channel 03.
Channel 03 was — and if you can dig up an old television set, still is — simply a signal that appears to us as “static” or “snow.” That “snow” you see on your television comes from a combination of all sorts of sources:
- thermal noise of the television set and its surrounding environment,
- human-made radio transmissions,
- the Sun,
- black holes,
- and all sorts of other directional astrophysical phenomena like pulsars, cosmic rays and more.
But if you were able to either block all of those other signals out, or simply took them into account and subtracted them out, a signal would still remain. It would only be about 1% of the total “snow” signal that you see, but there would be no way of removing it. When you watch channel 03, 1% of what you’re watching comes from the Big Bang’s leftover glow. You are literally watching the cosmic microwave background.
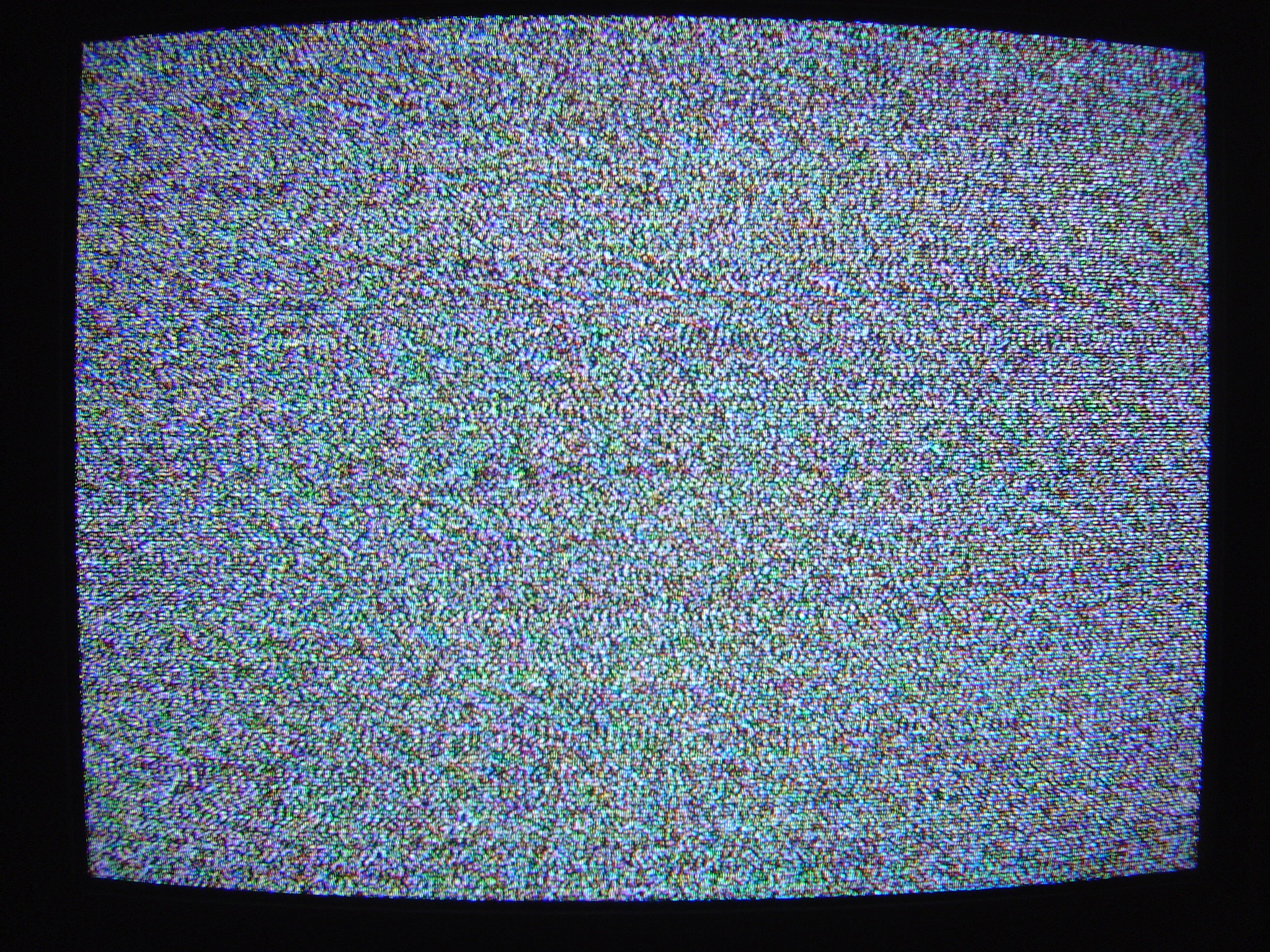
The “snow” you see on channel 03 on your television set is a combination of a variety of static-producing signals, most of which arise from human-made radio transmissions on Earth and from the Sun. But about 1% of the static we see is from the Big Bang’s leftover glow: the cosmic microwave background. Even in the deepest depths of intergalactic space, the Big Bang is still broadcasting.(Credit: Arnold Chao of arnisto.com; flickr)
For the ultimate cosmic experiment, consider activating an old-fashioned rabbit-ear-style television set on the secluded far side of the Moon. Here, it would be completely shielded from Earth's radio signals. Moreover, during lunar nighttime, it would also escape the full spectrum of the Sun’s radiation. Powering up this television and tuning it to channel 03, you'd observe a relentless snow-like signal, persisting even without any transmitted signals.
This residual static is irremovable, remaining unaltered in strength or signal quality regardless of how you adjust the antenna’s position. The explanation for this phenomenon is fascinating: the signal is actually emanating from the cosmic microwave background itself. By isolating the various sources causing the static and analyzing what remains, anyone post-1940s could have detected the cosmic microwave background at home, confirming the Big Bang theory years before official scientific validation.
In an era where we are constantly warned, "Don't try this at home," this forgotten technology is a reminder that some home experiments can unlock secrets of the universe.
You Might Also Like :
0 commenti:
Post a Comment